Study Shows How Stress Causes Extensive Genetic Rewiring
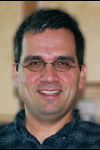
A team of scientists in the United States, South Korea, and Switzerland has uncovered a vast, complex network of 160,000 genetic interactions within yeast cells that changes dramatically when the cells are subjected to stress.
The “rewiring” of this genetic network is much more extensive than scientists previously thought. About 70 percent of the genetic interactions that took place when the cells were under stress did not take place in normal, unperturbed cells.
“The stress response is dynamic,” says Nevan Krogan, PhD, an associate professor of Cellular and Molecular Pharmacology at UCSF and an affiliate of the California Institute for Quantitative Biosciences (QB3). “In the cell, things are constantly changing and functional modules are being rewired.”
Krogan and Trey Ideker, PhD, chief of the Genetics Division at the UC San Diego School of Medicine, led the study, which was described in the journal Science last month, with first author Sourav Bandyopadhyay, PhD – a fellow at QB3 and the UCSF Helen Diller Family Comprehensive Cancer Center – and colleagues at Albert Einstein College of Medicine, Seoul National University in Korea, and ETH Zurich in Switzerland.
The new work has implicated several genes that were never before known to be involved in stress response, with immediate implications for scientists who study biological responses to stress, the authors say. The technique used also may prove useful for studying a wide variety of human diseases, by providing a new way of examining broadly how cells deal with stresses, diseases, drugs, or other challenges.
How a cell is wired genetically – the exact way its thousands of genes interact and “talk” to one another – is a critical issue for understanding the inner workings of the cell. In the last decade or so, the revolution in DNA sequencing has led to a wealth of new information about which genes are present and active in many types of cells.
Often this data is static, however, and is limited to information about which genes are present but not how these genes interact or how these interactions change over time. The difference is analogous to comparing a photograph with a video.
Imagine a busy playground full of children in the summer. A psychologist examining child behavior might find a photo of the playground useful. It would reveal the structures, the people, and perhaps many of the human interactions. But a video might reveal rich details not seen in the static image, such as which kids are playing with each other, which are playing by themselves, which adults seated on the benches are attentive, and which are distracted. A video might even reveal how a dramatic change to the environment, like the sound of an approaching ice cream truck, alters the children’s play. The same is true for the landscape of a living cell, says Krogan.
“What we’d really like to have,” he says, “is an animated movie.”
Up to now, however, there hasn’t been a way to grab more than a single snapshot with respect to large-scale interaction networks.
Now, using a new technique called differential epistasis mapping, Krogan and his colleagues have been able to take a step toward a more animated view of the dynamic rewiring inside cells. Using the technique, they have generated two snapshots of about 160,000 genetic interactions within yeast—one set of interactions that exists under normal conditions and one set that exists when the cells are stressed, having been exposed to a DNA-damaging chemical called methyl methanesulfonate.
The snapshots reveal how widely yeast cells rewire their genetic networks to deal with the DNA-damaging stress. In their article, Krogan and his colleagues detail how the technique helped them to identify sets of genes involved in the cell’s response to the DNA damage that were not previously known to be involved in DNA repair.
“Nobody could have predicted the connections we are finding in the presence of stress,” says Krogan.
The article, titled, “Rewiring of Genetic Networks in Response to DNA Damage,” appeared in the Dec. 2, 2010, issue of the journal Science and online at http://www.sciencemag.org/content/330/6009/1385.full.html.
Bandyopadhyay was affiliated with UC San Diego, La Jolla, CA, at the time of the research, as are co-authors Ryan Chuang, Wilbert Copeland, Janusz Dutkowski, Eric J. Jaehnig, Dwight Kuo, Richard D. Kolodner, Katherine Licon, and Ideker. Additional co-authors include Monika Mehta and Michael-Christopher Keogh, from Albert Einstein College of Medicine, Bronx, NY; Min-Kyung Sung and Won-Ki Huh, from Seoul National University, Seoul, Korea; Bernd Bodenmiller and Ruedi Aebersold, of the Institute of Molecular Systems Biology and University of Zürich, Switzerland; Michael Shales, Dorothea Fiedler, Kevan M. Shokat and Krogan, of UCSF; and Aude Guénolé, of Leiden University Medical Center, Netherlands. Fiedler and Shokat also are affiliated with the Howard Hughes Medical Institute, San Francisco. Full affiliations can be found in the published article.
The research was supported by grants from the National Institutes of Health, as well as the Frontier Functional Proteomics Project, the Swiss National Science Foundation, Keck Young Investigator fellowship, Searle fellowship and David and Lucille Packard fellowship. Additionally, Kolodner is a paid consultant to On-Q-ity. The authors declare no conflicts of interest in this work.
UCSF is a leading university dedicated to promoting health worldwide through advanced biomedical research, graduate-level education in the life sciences and health professions, and excellence in patient care. For more information, visit www.ucsf.edu.